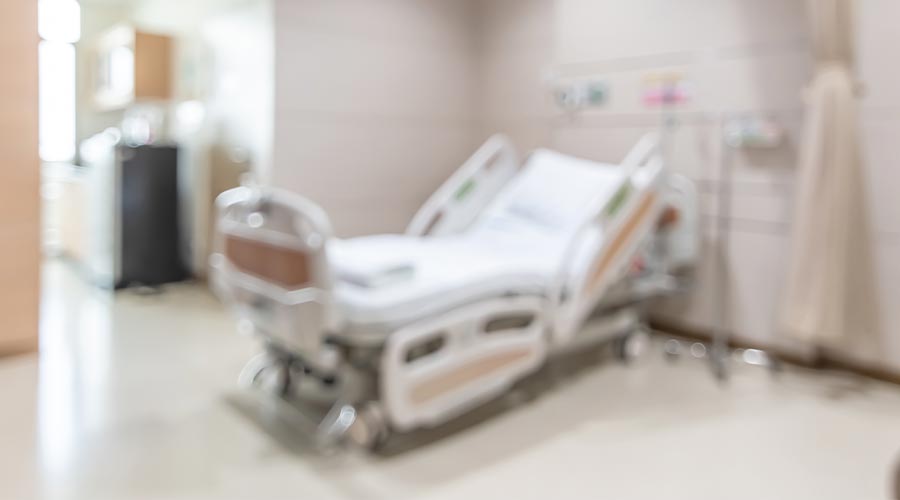
By Dr. Charles P. Gerba and Dinesh Wadhwani
A version of this article was originally published by Infection Control Today. Republished with permission.
The Richter Scale developed by Charles F. Richter in 1935 used seismograph technology to measure the strength of earthquakes on a logarithmic scale from one to 8.6 (the largest earthquake on record) (Rafferty, 2024). It made complicated data simple to grasp through an at-a-glance index of magnitude to help public health and safety officials anticipate impacts.
Richter’s scale was largely replaced by the Moment Magnitude Scale in the late 1970s (Ranjit Das, 2024), and advances in seismograph technology enabled early warning systems such as the U.S. Geological Service (USGS) ShakeAlert that detects the rumblings of potentially large temblors and sends alerts to communities before major shaking starts. (USGS, 2017)
Standardized, more sensitive and accurate ways of measuring earthquakes improve our ability to cope with related impacts and exposures.
Advances in IAQ monitor technology (see Sidebar: "Technological Advancements in IAQ Monitors") suggest a scale that helps anticipate IAQ impacts by indexing levels of exposure in real-time; e.g., wall-mounted units can detect tremors of exposure to 0.1-micron particles linked to viral load and transmission based on particle size and shape profiling (see Sidebar: “The Role of Shape in Identifying Microscopic Particles as Viruses”) a heightened sensitivity that extends to volatile organic compounds (VOCs), chemical and other pollutants enhanced by plug-and-play modules that detect certain contaminant types.
Like a supercharged Richter Scale, an IAQ Exposure Index (INDOOR AIR QUALITY EXPOSURE INDEX) — as a complement to EPA’s outdoor Air Quality Index or AQI — can help:
1. Determine the scope of airborne exposures using a simple at-a-glance metric,
2. Provide an early warning system, and
3. Remediate indoor air by better managing airflow, ventilation, and purification to reduce infectious illness and other exposures.
The Need in Healthcare
Healthcare facilities serve the needs of high-risk individuals — such as chemotherapy patients, the immune-compromised, those with respiratory conditions, neonates, and the elderly — who are most susceptible to airborne exposures. Healthcare work also tops the list of high-proximity jobs, those involving close contact with others. Thus, a scale that provides an instant exposure metric of airborne pollutants may have seismic impacts in this vulnerable community.
Placement of sensors is critical due to the way air moves through indoor spaces, and since air is “liquid” — understanding widening exposures from an ocean oil-spill helps convey the movement of pollutants in indoor air.
Understanding Airborne Exposures
In an oil spill, petroleum particles spread into and permeate the environment. Cleanup involves not only removing what you can see (e.g., globs of oil) but unseen particles and gaseous pollutants wafted on currents that may be the most harmful over time as they often escape detection and removal. Well-placed optical sensors, electrochemical sensors, ultrasonic sensors and conductivity sensors are used to detect oily particles and byproducts when remediating a spill (Rika Sensor, 2023).
Well-placed air sensors help detect what’s in the ocean of indoor air (see Sidebar: Common Air Pollutants in Healthcare Facilities), and from an infection prevention perspective; viruses, bacteria, and fungi which may circulate in air attached to other particles, Trojan-horse style (Tellier, 2006). (see Sidebar: Airborne Pathogens 0 .1 Micron or Larger).
An Early Warning System for IAQ
As a seismograph detects even slight and unusual increases of ground motion in real-time, providing an early-warning system for seismologists, a system driven by an INDOOR AIR QUALITY EXPOSURE INDEX can alert IPC and EVS staff of potential IAQ issues and spikes.
Continuous monitoring of air quality — and, say, providing a one to 10 metric for airborne viral load — will help enable early detection and intervention as a surveillance system to spot outbreaks and facilitate prompt responses to mitigate the spread of infections.
By identifying and quantifying levels of airborne pollutants, an INDOOR AIR QUALITY EXPOSURE INDEX can place the focus on eliminating sources of pollutants where possible, better managing airflows, improving ventilation, and integrating air purification with building management systems (Vrushank Mistry, 2023).
A system to reduce airborne-source infections is best summed up by a three-circle system of air monitoring, ceiling-mount and floor-based air purification, based on the principle that purifiers closer to the breathing zone of occupants are most effective (Cheryl K DuBois , Michael J Murphy , Amanda J Kramer , Jodi D Quam, Andrew R Fox , Ty J Oberlin, Perry W Logan, April) (See Sidebar: “Three-Circle Venn Diagram: Air Monitoring and Purification”).
Remediate by Managing Airflow, Ventilation, and Purification
Since air — though life-giving — is a carrier of pollutants, controlling the air through room pressurization and directing airflow is vital but insufficient since infectious exposure often occurs in proximity to the source before contaminated air can be removed, filtered and diluted by HVAC systems.
Room- or area-based sensors integrated with ceiling-mounted or freestanding air purifiers enable crucial proximity air cleaning to intercept pollutants at the source, protect the immediate exposure zone, and lower HVAC costs since cleaner air reaching the HVAC system reduces filter changes, electricity for operating cycles, and motor wear.
Conclusion
Success in protecting air quality based on an INDOOR AIR QUALITY EXPOSURE INDEX involves an at-a-glance metric to make identifying complex airborne exposures simpler, provides an early warning system for airborne pathogens, and lowers costs for HVAC, all while producing a seismic shift in protecting human health by integrating monitoring (diagnosis) and purification (treatment) in real time to improve the indoor air in healthcare facilities.
Sidebar: Technological Advancements in IAQ Monitors
The concept of monitoring indoor air quality (IAQ) began taking shape in the late 20th century, driven by increasing awareness of the health impacts of indoor pollutants. The first IAQ monitors were rudimentary devices that primarily measured particulate matter and basic gases such as carbon dioxide (CO2) and carbon monoxide (CO). These early monitors provided limited data and required manual reading and recording, often making them cumbersome and less effective for continuous monitoring.
The evolution of IAQ monitors has been marked by numerous technological advancements, making today's devices more accurate, user-friendly, and versatile. These advancements include improvements in sensor technology, integration with smart technologies, and enhanced data analytics capabilities.
Sensor Technology
Modern IAQ monitors use advanced sensor technology that allows for the detection of a broader range of pollutants with greater precision. Sensors have become more sensitive and can now measure fine particulate matter (PM2.5 and PM10), volatile organic compounds (VOCs), humidity, temperature, and other parameters. Remarkably, some units can detect 0.1 micron sized particles and particle shapes promising an early warning system for viral exposure (see Sidebar: “The Role of Shape in Identifying Microscopic Particles as Viruses”).
The use of cloud computing and advanced data analytics has further revolutionized IAQ monitoring. Data collected by IAQ monitors can now be stored and analyzed over time, providing valuable insights into airflow patterns and trends in air quality.
An INDOOR AIR QUALITY EXPOSURE INDEX can predict potential air quality issues and suggest or initiate proactive steps — e.g., ventilation, directed airflow, and sensor-integrated purification — to protect human health.
Sidebar: The Role of Shape in Identifying Microscopic Particles as Viruses
Viruses come in various shapes, each reflecting their unique structures. Here are the primary shapes:
Icosahedral
Think of Adenoviruses. They have an icosahedral shape, which means they have 20 triangular faces. This shape provides stability and symmetry. (Solène Besson, 2020)
Helical
Consider the Tobacco mosaic virus. It has a rod-like shape, formed by protein subunits spiraled around a central axis.
Complex
Look at bacteriophages. These viruses are complex, combining icosahedral and helical features, often with added structures like tails.
Summing Up
The shape of microscopic particles is crucial in identifying them as viruses.
Using advanced techniques like electron microscopy, X-ray crystallography, and Cryo-EM, scientists can see and differentiate these pathogens based on their unique forms. This knowledge is vital for diagnostics, vaccine development, and tracking outbreaks.
Sophisticated air monitoring systems can now also help identify viral shapes, ultimately helping us understand distribution patterns and prevent viral infections.
Sidebar: Common Air Pollutants in Healthcare Facilities
Particulate Matter (PM)
Particulate matter refers to a mixture of airborne solid particles and liquid droplets. These particles when inhaled can impact health. Sources of PM in healthcare facilities include:
• Linens and bedding generate fine textile dust.
• Skin flakes from patients and staff. An average person sheds about 600,000 skin flakes every day or 1.5 pounds of skin cells shed per year [Texas A&M University].
• Indoor Activities: Certain medical procedures and laboratory activities can produce particulate matter.
• Construction Activities: Renovation and construction work within healthcare facilities can generate dust and other particulate matter.
• Outdoor Air: Particulate matter from outside can infiltrate the building through ventilation systems.
Pathogens
In healthcare settings, airborne pathogens are a significant concern, including:
• Bacteria
• Viruses
• Fungi: Molds and other fungi can grow in damp areas and release spores that may cause allergic reactions and infections.
Volatile Organic Compounds (VOCs)
VOCs are chemicals that become airborne as vapors or gases. They are released from various sources within healthcare facilities, including:
• Cleaning Agents: Many disinfectants and cleaning solutions emit VOCs which can contribute to respiratory issues and other health problems.
• Medical Equipment, Materials: Some medical devices and materials can release VOCs.
• Building Materials: Paints, adhesives, and synthetic materials used in the construction and maintenance of healthcare facilities often emit VOCs.
Chemical Contaminants
Healthcare facilities often use various chemicals that can contaminate the air, including:
• Disinfectants and Sterilants
• Pharmaceutical Compounds
Gaseous Pollutants
Gases such as carbon monoxide (CO) and nitrogen dioxide (NO2) can originate from:
• Combustion Processes: Heating systems, generators, and other combustion sources can emit harmful gases.
• Medical Gas Systems: Leaks and emissions from medical gas systems can introduce pollutants into the indoor air.
Sidebar: Airborne Pathogens 0.1 Micron or Larger
-
Influenza Virus: Commonly known as the flu, it can be spread through respiratory droplets.
-
Rhinoviruses: These are a major cause of the common cold.
-
Adenoviruses: Can cause respiratory, gastrointestinal, and other infections.
-
Respiratory Syncytial Virus (RSV): A common cause of respiratory infections, especially in young children and older adults.
The sizes of some airborne bacteria in microns are as follows:
-
Staphylococcus bacteria (commonly known as staph) are generally between 0.5 to 1.5 microns in diameter.
-
Pseudomonas aeruginosa: Typically, these bacteria measure around 1-2 microns in diameter and 5-8 microns in length.
-
Mycobacterium tuberculosis: These bacteria are about 0.2-0.5 microns in diameter and 2-4 microns in length.
-
Acinetobacter baumannii: Generally, they are roughly 0.9-1.6 microns in diameter.
-
Legionella pneumophila: These bacteria are approximately 0.5-0.7 microns in width and 1-3 microns in length.
(Fennelly, 2020)
Sidebar: Three-Circle Venn Diagram: Air Monitoring and Purification
An integrated approach to diagnosing and treating indoor air best is described by a three-circle Venn chart.
Three-Circle Venn Diagram: Air Purification and Monitoring
This Venn diagram explores how three essential elements of air purification and monitoring intersect:
Effective Air Monitoring
• Collects real-time data
• Analyzes air quality
• Detects pollutants and allergens
Ceiling Mount Air Purifiers
• Permanently installed
• Cover large areas
• Operate quietly
Rolling Floor Air Purifiers
• Are portable
• Position flexibly
• Clean the air in a targeted approach
Intersections
Effective Air Monitoring and Ceiling Mount Air Purifiers mean continuous air quality control combined with high-efficiency purification for large spaces.
Effective Air Monitoring and Rolling Floor Air Purifiers provide real-time air quality feedback with mobile air cleaning solutions for adaptable environments.
Ceiling Mount Air Purifiers and Rolling Floor Air Purifiers offer a comprehensive air purification strategy utilizing both fixed and portable units for maximum coverage.
Combining all three — Effective Air Monitoring, Ceiling Mount Air Purifiers, and Rolling Floor Air Purifiers — gives you an integrated system to achieve 4-log purification (99.99 percent) of airborne particles, pathogens and their carriers, down to 0.1 micron.
This setup offers extensive air quality monitoring and versatile purification options, ensuring optimal indoor air quality in any setting.
Authors
Charles P. Gerba PhD
Professor of Environmental Microbiology, University of Arizona.
Charles P. Gerba, PhD is an internationally recognized environmental microbiologist and Professor of Environmental Microbiology in the Departments of Microbiology and Immunology, and Soil, Water and Environmental Science, at the University of Arizona. His credentials include a BA in Microbiology, Arizona State University, 1969, and a PhD in Microbiology, University of Miami, Florida, 1973. He is also a member of the American Academy of Microbiology.
Dinesh Wadhwani
Dinesh Wadhwani is an industry, air quality and lighting expert, entrepreneur in the technology and life sciences industries, and Founder/CEO of ThinkLite.
At ThinkLite, he works with a team of engineers to create “high value add” technological solutions in diverse fields including pharmaceutical, agriculture, poultry, data centers, general health and most recently, a technology solution that tracks the levels of airborne pathogens in indoor public areas and facilities, including COVID-19, among other viruses; so they can be addressed and managed to help optimize health and safety indoors.
References
Cheryl K DuBois , Michael J Murphy , Amanda J Kramer , Jodi D Quam, Andrew R Fox , Ty J Oberlin, Perry W Logan. (April, 2022 8). Use of portable air purifiers as local exhaust ventilation during COVID-19 . Retrieved from PubMed and Journal of Occupational and Environmental Hygiene: https://pubmed.ncbi.nlm.nih.gov/35290164/
Fennelly, K. P. (2020, September ). Particle sizes of infectious aerosols: implications for infection control. Retrieved from The Lancet: https://www.thelancet.com/journals/lanres/article/piis2213-2600(20)30323-4/fulltext
Rafferty, J. P. (2024, October 19). Richter scale. Retrieved from Encyclopedia Brittanica: https://www.britannica.com/science/Richter-scale
Ranjit Das, M. L. (2024, March 28). Comment on “A Seismic Moment Magnitude Scale”. Retrieved from Bulletin of the Seismological Society of America: https://pubs.geoscienceworld.org/ssa/bssa/article-abstract/114/4/2270/637047/Comment-on-A-Seismic-Moment-Magnitude-Scale-by?redirectedFrom=fulltext
Rika Sensor. (2023, August 7). What Sensor Can I Use To Detect Oil In Water? Retrieved from Rika Sensor: https://www.rikasensor.com/what-sensor-can-i-use-to-detect-oil-in-water.html
Solène Besson, C. V. (2020, July). The Adenovirus Dodecahedron: Beyond the Platonic Story. Retrieved from ResearchGate: https://www.researchgate.net/publication/342687794_The_Adenovirus_Dodecahedron_Beyond_the_Platonic_Story
Tellier, R. (2006, November). Review of Aerosol Transmission of Influenza A Virus. Retrieved from CDC: https://wwwnc.cdc.gov/eid/article/12/11/06-0426_article
USGS. (2017, April 6). “ShakeAlert” Earthquake Early Warning System Goes West Coast Wide. Retrieved from USGS.gov: https://www.usgs.gov/news/state-news-release/shakealert-earthquake-early-warning-system-goes-west-coast-wide
Vrushank Mistry. (2023, December). Impact of Building Automation on Indoor Air Quality and HVAC Performance. Retrieved from ResearchGate: https://www.researchgate.net/publication/378416265_Impact_of_Building_Automation_on_Indoor_Air_Quality_and_HVAC_Performance
posted on 4/11/2025